The third in a series examines powerful new gene drive tools
Originally published at Legal Planet.
In my previous two posts, I introduced what I call first, second, and third generation genetically modified organisms: (1) GM bacteria for diverse, mostly indoor purposes; (2) GM crops and agricultural animals; and (3) GMOs that would be intentionally placed into natural environments, where they would live, reproduce, and transmit their modified genes to offspring.
A limitation with the final category is that an entire wild population (that is, those organisms within a local ecological community) cannot be readily genetically modified. After GMOs’ introduction, their novel genes and traits would spread somewhat through reproduction with wild organisms and then disappear due to natural selection. This is because most traits that humans might add would not give wild organisms a reproductive advantage and most would confer a disadvantage. After all, if a change were advantageous, then the trait likely would have evolved on its own. In short, it is difficult for biotechnology to compete with millions of years of natural selection. Thus, genetically modifying a wild population through third generation techniques requires repeatedly releasing large numbers of GMOs, which is possible, but expensive and impermanent.
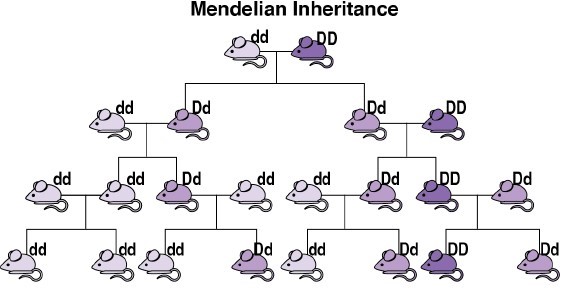
Scientists have developed a tool that could overcome this limitation. Understanding these requires a quick review of genetics. In sexually reproducing species, an organism typically has equal chances of transmitting to its offspring the version of a gene that it got from either its male parent or its female parent (see image). In 2003, Austin Burt noted that, in some uncommon natural instances, one of the sets of an organism’s gene has a greater chance of being transmitted than the other. He proposed that humans could synthesize similar mechanisms of biased inheritance to “drive” a desired modification gene through a wild population. Burt’s proposal remained largely theoretical until the development of CRISPR-based gene editing in 2012, enabling CRISPR-based gene drive systems (usually called simply “gene drives”).
In these, a gene drive copies itself and an accompanying modified genetic sequence onto the equivalent location on the other set of genes, causing them to be transmitted to all of an individual’s offspring (see image). This way, gene drives empower humans to genetically modify an entire wild population by introducing only a small number of GMOs and then waiting a few generations. These could spread a desired variant of a gene, reduce the size of population, or extinguish it entirely. Importantly, gene drives are effective only in species that reproduce sexually and have a short life cycle, as several generations are necessary before most of a population becomes altered. This excludes their use in bacteria and large animals, such as humans. Researchers have successfully modified species such as yeast, fruit flies, and mosquitoes to carry and propagate gene drives, while initial steps with mice were recently successful. Gene drive organisms have been tested in contained environments, and some scientists have suggested that outdoor experiments could take place as early as 2023. (If you wish to learn more, the US National Academies of Science, Engineering, and Medicine published an essential report in 2016, although this is already becoming outdated.)
Like third generation GMOs, gene drives offer applications for both human well-being and biodiversity conservation:
- Most research to date has concerned combating disease that afflict humans. Malaria, dengue fever, Zika, chikungunya, Mayaro, and yellow fever are transmitted by only a few mosquito species (of which there are thousands). Populations of them could be reduced or eliminated in areas where the diseases are endemic. Alternatively, gene drives could modify populations so that the vector species could not carry, transmit, or propagate the disease.
- Gene drives could benefit agriculture. Pest populations could be reduced, eliminated, or modified to remove their evolved resistance to insecticide. Along similar lines, gene drives could make help protect species that are negatively affected by pesticides but not targeted.
- Endangered species could be genetically modified to resist diseases. For example, there is growing evidence that a fungus is the primary cause of the recent decline in amphibian populations, which could be modified to be unaffected by it.
- Humans could genetically modify wild populations to increase resilience in the face of changing conditions, particularly due to anthropogenic climate change.
- Gene drives could reduce or extirpate invasive alien species, one of the leading drivers of biodiversity loss. This is particularly appealing in islands, which are more vulnerable to invasive aliens and could better contain a gene drive. Alternatively, a gene drive could modify an invasive alien species to be less competitive with native ones. For example, ragweed, which is invasive in many areas outside of North America, could be made shorter.
New Zealand offers a particularly interesting case where gene drives could be used to help conserve biodiversity. A set of relatively isolated islands that were among the last to be settled by humans, it is a biodiversity hotspot with “extremely high” terrestrial species endemism (the condition of being unique to a locality). Before humans arrived approximately 700 years ago, the only terrestrial mammals were bats. This lack of predators caused native species to lose through evolution many of their defenses against predation. For example, some bird species became flightless. Human settlement – especially by Europeans – brought numerous invasive alien species, including several dozen terrestrial mammals, some of which are predators. Now, after almost sixty extinctions, about one-third of native bird species are threatened and another half are at risk [PDF]. Among these are most species of kiwi, an unofficial national symbol. Invasive alien predators are the primary driver of loss. In 2016, the government resolved that New Zealand will be free of the most harmful alien predators – rats, stoats, weasels, ferrets, and possums – by 2050. Some scientists believe that “predator-free New Zealand is possible through use of novel ‘next-generation’ pest control tools,” and gene drives have been among the tools being researched to eradicate invasive rats and wasps [PDF].
GMOs with gene drives would pose environmental risks and social challenges. Their outdoor use could affect target organisms’ genomes at other locations, populations of the target species beyond the desired one, or non-target species via horizontal gene transfer. The desired change could have unexpected impacts on ecosystems. Because of these risks, gene drives’ indoor experimental use also presents concerns of accidental release. In terms of social issues, gene drives’ research, use, and governance are deeply value-laden, and modifying or eradicating populations presents challenging ethical questions. It is unclear who has the legitimate authority to introduce gene drives organisms. Proposals to use gene drives to help conserve biodiversity could perhaps weaken efforts to do so by other means, such as by preserving critical habitat. Finally, gene drives could also be used for malicious purposes, at least in principle.
Although these risks should be taken seriously, some might be less severe than they initially seem. Horizontal gene transfer from animals is quite rare. Any ecological impacts of eradicating species are independent of whether this is done through gene drives or more traditional techniques. Furthermore, scientists believe that removing a single mosquito species would not be ecologically harmful. Populations are also likely to evolve resistance to population-reducing drives, limiting their effects. Gene drives may also be able to be technologically contained. For example, a “daisy chain” drive could only be transmitted to offspring a limited number of times, allowing for localized applications. Likewise, an isolated population, such as those on islands, is often genetically distinct from other populations; a “precision” drive could be designed to affect only its members. A “threshold” gene drive system would be effective only if it were released above some frequency within the population, and would otherwise be driven out. Also, the spread of a gene drive to undesired populations may be able to be halted by the ex ante use of an “immunizing” one or remediated by the ex post introduction of a “reversal” one. Finally, these risks must be considered in the context of the countervailing ones, which include the loss of biodiversity through invasive alien species, the use of toxins to eradicate such species and other pests, and diseases among humans.
Considering the potential benefits and risks of this fourth generation of biotechnology, governance is critical. In the next post in this series, I will summarize how international legal institutions have responded to the proposed use of GMOs for the conservation of biodiversity.